The Future of Solar Paint Technology: Innovations, Challenges, and Market Potential
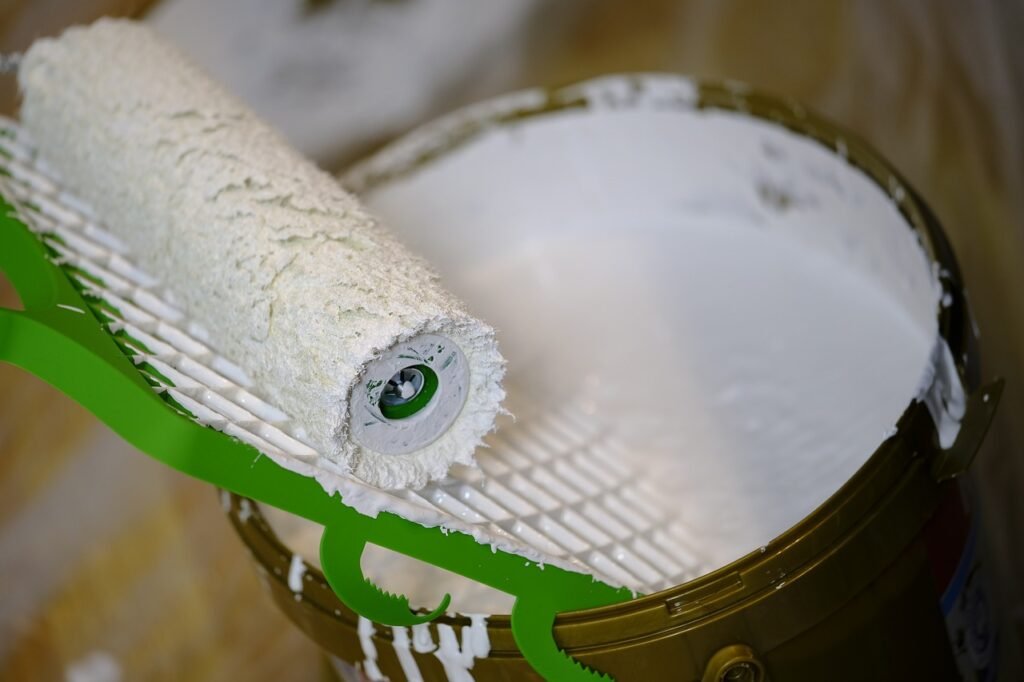
Executive Summary
Solar paint, an innovative photovoltaic technology, promises a cost-effective and versatile alternative to traditional silicon-based solar panels. This white paper explores the current state of solar paint technology, highlighting key innovations in materials such as perovskite, quantum dots, dye-sensitized solar cells, and hydrogen-generating solar paints.
While offering advantages like low production costs and flexible application, solar paints face challenges including stability, toxicity, and scalability. The paper assesses the commercialisation status, market trends, and future opportunities, providing insights for industry professionals, potential investors, and R&D collaborators in the chemistry and renewable energy sectors. Despite existing limitations, ongoing research and development efforts are paving the way for solar paint to play a significant role in the future of renewable energy.
Introduction
Solar paint, also known as paint-on solar or paintable solar, converts sunlight into electricity using light-sensitive materials suspended in a flexible liquid. This technology offers several advantages over traditional silicon-based solar panels, including lower production costs, simpler installation, and the ability to apply it to various shapes and sizes of surfaces.
Solar paint can be applied to numerous surface types including plastic, fabrics, and even car bodies. As the demand for renewable energy solutions grows, solar paint has the potential to transform the energy market by making solar power more accessible and versatile.
Key Innovations
Perovskite Solar Cells
Perovskite solar cells (PSCs) are a promising technology in the field of solar paint due to their high power conversion efficiency and relatively easy manufacturing.
- Materials and Structure: Perovskites are materials with a crystal structure similar to calcium titanate (CaTiO3), following the formula ABX3, where A and B are cations, and X is an anion. The most common absorber material is methyl ammonium lead trihalide (MAPbI3), known for its high absorption coefficient and a bandgap close to the ideal 1.3 eV for solar cells. PSCs typically consist of five components: a metal-based cathode, a hole transport layer (HTL), an absorber layer (AL), an electron transport layer (ETL), and a transparent conductive oxide (TCO).
- Progress in Device Architecture: The power conversion efficiency (PCE) of PSCs has rapidly increased from 3.8% to 25.2% through modifications in device architecture. Key architectures include:
- Dye-sensitized structure: The initial configuration with low efficiency (3.8%).
- Solid-state mesoscopic structure: Replacing the liquid electrolyte with a solid hole conductor improved efficiency and stability.
- Meso-superstructure: Replacing m-TiO2 with an insulating layer of m-Al2O3 increased PCE up to 10.9%.
- Planar n-i-p heterojunction structure: Achieved a PCE of 21.6% using EDTA complex tin oxide (SnO2) as ETL and FAPbI3 as the absorber layer.
- Inverted planar structure: This configuration has a reverse sequence of ETLs and HTLs from the regular structure, and attained an average PCE of 15.47%.
- Film Fabrication Techniques:
- Single-step deposition: The precursor solution is spin-coated over the TiO2 or SnO2 scaffold.
- Two-step sequential deposition: A film is deposited and then converted to a perovskite layer upon reaction with methylammonium iodide (MAI) solution.
- Inkjet printing: Offers mask-less on-demand patterning, low cost, and efficient use of material.
- Doctor blade coating: A fixed amount of perovskite precursor solution is poured over the substrate, followed by swiping the glass blade linearly with a high speed.
- Spray coating: Involves dispersing droplets over a substrate using ultrasonic vibration, fast gas flow or electrostatic repulsive force.
Quantum Dot Solar Cells

Quantum dot solar cells (QDSCs) utilise quantum dots (QDs) as the absorbing photovoltaic material. QDs are nanoscale crystals that transport electrons and emit light of various colours when exposed to sunlight.
- Materials and Properties: Quantum dots possess a tunable bandgap, multiple exciton generation (MEG), and higher relaxation time. Common materials include CdX, ZnX, PbX, SnX (X=S, Se, Te), HgTe, InP, InAs, GaAs, GaP, and CuInS2. Eco-friendly QDs like carbon and graphene QDs are also being explored.
- Device Structure:
- Schottky QDSCs: The QD active layer is sandwiched between a transparent electrode and a counter metal electrode.
- Depleted heterojunction QDSCs: QD layers are deposited on wide bandgap energy oxides (TiO2 or ZnO), forming junctions with the electron transport layers (ETLs).
- Quantum junction QDSCs: N-type QDs and p-type QDs are used as a heterojunction to extend the depletion region at the junction area.
- Quantum dot sensitized solar cells (QDSCs): Consist of wide bandgap metal oxide (TiO2, ZnO, and SnO2) as photoanode, inorganic semiconductor QD deposited on photoanode as light sensitizer, liquid polysulfide electrolyte and counter electrode.
- Improving QD Film:
- Ligand exchange and QD passivation: Replacing long-chain ligands with short ones to improve film conductivity.
- Multiple exciton generation (MEG): Utilising high energy to generate additional electron-hole pairs.
- Film Fabrication Techniques:
- Drop casting: A liquid solution drop is spread over the target area, and the solvent completely evaporates.
- Spin and dip coating: A QD solvent is spread on a substrate that changes into a thin film through high-speed rotation.
- Inkjet printing: Deposits material with reduced material consumption over a target area.
- Spray coating: A QD solution is sprayed through a nozzle using high pressurised gas.
- Doctor-blade or Slot-die coating: A uniformly coated layer can be achieved in slot-die and/or doctor-blade coating.
Dye-Sensitized Solar Cells
Dye-sensitized solar cells (DSSCs) are cost-effective photovoltaic devices known for inexpensive materials and simple fabrication.
- Components:
- Transparent and conductive substrate: Requires more than 80% transparency and high electrical conductivity.
- Working electrode (WE): Prepared by depositing a thin layer of oxide semiconducting materials on a transparent conducting glass plate.
- Photosensitizer or dye: Responsible for the maximum absorption of incident light.
- Electrolyte: Contains a redox couple, solvent, additives, ionic liquids, and cations.
- Counter electrode (CE): Catalyses the reduction of liquid electrolyte and collects holes from the hole transport materials (HTMs).
- Working Principle: The DSSC working principle involves four basic steps: light absorption, electron injection, transportation of carrier, and collection of current.
Solar Paint Hydrogen

This type of solar paint produces hydrogen by absorbing moisture from the air and using solar energy to split water molecules.
- Materials: Titanium dioxide is paired with synthetic molybdenum disulphide, which facilitates sustainable solar to hydrogen conversion under visible light irradiation.
- Mechanism: Molybdenum disulphide takes energy from titanium dioxide, pulls water molecules from the air, and splits them into hydrogen and oxygen.
- Advantages: No need for clean or filtered water; can be used in remote areas with water vapour in the air.
Challenges
Technical Challenges
- Stability: Perovskite materials degrade when exposed to moisture, oxygen, light, and heat. Quantum dots are susceptible to oxygen, light, and thermal degradation. Electrolyte degradation in DSSCs affects cell efficiency.
- Toxicity: The presence of lead in perovskite materials and some quantum dots poses environmental and health risks.
- Efficiency: Although perovskite solar cells have high efficiency, quantum dot solar cells still require better understanding of their energy level, poor size control, and lack of a good synthesis method to improve their efficiency. The efficiency of carbon-based counter electrode DSSCs is lower than that of a cell fabricated by Pt-based counter electrode. The hydrogen production rate of solar paint hydrogen declines upon prolonged illumination.
- Scalability: Scaling up production while maintaining performance is a challenge for all types of solar paints.
Environmental Challenges
- Lead Toxicity: Perovskite solar cells typically contain lead, a neurotoxin, raising environmental concerns.
- Material Disposal: Safe disposal and recycling methods are needed to handle solar paint materials at the end of their life cycle.
Commercialization Challenges
- Cost-Effectiveness: Balancing the cost of materials and manufacturing processes to compete with existing solar technologies is crucial.
- Market Acceptance: Overcoming the perception that solar paint is less efficient or durable than traditional solar panels.
- Regulatory Hurdles: Meeting industry standards and obtaining certifications for solar paint products.
Market Potential
Commercialization Status
- Perovskite Solar Cells: Companies like Oxford PV have achieved high efficiencies in perovskite-silicon tandem cells. Solliance has demonstrated large perovskite thin-film modules on glass.
- Quantum Dot Solar Cells: NREL has developed QDSCs with 13.4% conversion efficiency. The University of Queensland achieved 16.6% efficiency using halide perovskites.
- Dye-Sensitized Solar Cells: Companies like Solaronix and Dyesol have made progress in commercial production. IIT Roorkee and CSIR-NCL have developed organic dyes to enhance DSSC efficiency.
- Market Trends: The dye-sensitized solar cell market was valued at USD 90.5 million in 2019 and is expected to grow at a CAGR of 12.4% from 2020 to 2027.
Future Opportunities
- Building Integrated Photovoltaics (BIPV): Solar paint can be integrated into building materials, offering a cost-effective way to generate electricity.
- Portable Electronics: Flexible solar paint can power portable devices, reducing reliance on traditional batteries.
- Automotive Industry: Applying solar paint to car bodies can supplement electric vehicle power, increasing driving range.
- Remote Power Generation: Solar paint can provide electricity in remote areas where traditional power sources are not readily available.
Conclusion

Solar paint technology represents a promising frontier in renewable energy, offering numerous advantages and the ability to transform various sectors. While significant challenges related to stability, toxicity, and scalability remain, ongoing research and development efforts are steadily improving the technology.
With continued innovation and strategic market positioning, solar paint has the potential to become a significant player in the global renewable energy landscape, offering a versatile and accessible solution for a sustainable future.
Partner with Us
We are a technical consulting solutions and custom product development consultancy. Get in touch with us to get a free introductory session with us for project scoping.
References
- Boschloo, Gerrit. “Improving the performance of dye-sensitized solar cells.” Frontiers in chemistry 7 (2019): 77.
- Khan, Shaheer A., and Ataur Rahman. “Efficiency of thin film photovoltaic paint: A brief review.” Int. J. Recent Technol. Eng 7 (2019): 163-169.
- Abbas, Muhammad A., Muhammad A. Basit, Seog Joon Yoon, Geun Jun Lee, Moo Dong Lee, Tae Joo Park, Prashant V. Kamat, and Jin Ho Bang. “Revival of solar paint concept: air-processable solar paints for the fabrication of quantum dot-sensitized solar cells.” The Journal of Physical Chemistry C 121, no. 33 (2017): 17658-17670.
- Semonin, Octavi E., Joseph M. Luther, and Matthew C. Beard. “Quantum dots for next-generation photovoltaics.” Materials today 15, no. 11 (2012): 508-515.
- Lee, Hyunho, Hyung-Jun Song, Moonsub Shim, and Changhee Lee. “Towards the commercialization of colloidal quantum dot solar cells: perspectives on device structures and manufacturing.” Energy & Environmental Science 13, no. 2 (2020): 404-431.
- Shi, Zhengqi, and Ahalapitiya H. Jayatissa. “Perovskites-based solar cells: A review of recent progress, materials and processing methods.” Materials 11, no. 5 (2018): 729.
- Carey, Graham H., Ahmed L. Abdelhady, Zhijun Ning, Susanna M. Thon, Osman M. Bakr, and Edward H. Sargent. “Colloidal quantum dot solar cells.” Chemical reviews 115, no. 23 (2015): 12732-12763.
- Zhou, Di, Tiantian Zhou, Yu Tian, Xiaolong Zhu, and Yafang Tu. “Perovskite-based solar cells: materials, methods, and future perspectives.” Journal of Nanomaterials 2018 (2018).
- Roy, Priyanka, Numeshwar Kumar Sinha, Sanjay Tiwari, and Ayush Khare. “A review on perovskite solar cells: Evolution of architecture, fabrication techniques, commercialization issues and status.” Solar Energy 198 (2020): 665-688.
- Fakharuddin, Azhar, Rajan Jose, Thomas M. Brown, Francisco Fabregat-Santiago, and Juan Bisquert. “A perspective on the production of dye-sensitized solar modules.” Energy & Environmental Science 7, no. 12 (2014): 3952-3981.
- Wei, Huiyun, Dongmei Li, Xinhe Zheng, and Qingbo Meng. “Recent progress of colloidal quantum dot based solar cells.” Chinese Physics B 27, no. 1 (2018): 018808.
- Daeneke, Torben, Nripen Dahr, Paul Atkin, Rhiannon M. Clark, Christopher J. Harrison, Robert Brkljača, Naresh Pillai et al. “Surface water dependent properties of sulfur-rich molybdenum sulfides: electrolyteless gas phase water splitting.” ACS nano 11, no. 7 (2017): 6782-6794.
- Hassan, Arhum, Mohsin Muhyuddin, Abdul Rahman, Muhamad Usman, Muhammad Abdul Basit, and Syed Wilayat Husain. “Improved optical and electrochemical performance of MoS2-incorporated TiO2-PbS nanocomposite for solar paint application.” Journal of Materials Science: Materials in Electronics 31, no. 3 (2020): 2625-2633.
- López-Rojas, Omar, and Jesús García Guzmán. “A review on quantum dot solar cells: properties, materials, synthesis and devices.” In 2019 IEEE International Conference on Engineering Veracruz (ICEV), vol. 1, pp. 1-5. IEEE, 2019.
- Mozaffari, Samaneh, Mohammad Reza Nateghi, and Mahmood Borhani Zarandi. “An overview of the Challenges in the commercialization of dye sensitized solar cells.” Renewable and Sustainable Energy Reviews 71 (2017): 675-686.
- Mora‐Seró, Iván. “Current Challenges in the Development of Quantum Dot Sensitized Solar Cells.” Advanced Energy Materials 10, no. 33 (2020): 2001774.
- Gong, Jiawei, K. Sumathy, Qiquan Qiao, and Zhengping Zhou. “Review on dye-sensitized solar cells (DSSCs): Advanced techniques and research trends.” Renewable and Sustainable Energy Reviews 68 (2017): 234-246.
- Sharma, Khushboo, Vinay Sharma, and S. S. Sharma. “Dye-sensitized solar cells: fundamentals and current status.” Nanoscale research letters 13, no. 1 (2018): 1-46.
- Kong, Fan-Tai, Song-Yuan Dai, and Kong-Jia Wang. “Review of recent progress in dye-sensitized solar cells.” Advances in OptoElectronics 2007 (2007).
- Baxter, Jason B. “Commercialization of dye sensitized solar cells: Present status and future research needs to improve efficiency, stability, and manufacturing.” Journal of Vacuum Science & Technology A: Vacuum, Surfaces, and Films 30, no. 2 (2012): 020801.
- Bolton, James R. “Solar photoproduction of hydrogen: a review.” Solar energy 57, no. 1 (1996): 37-50.
Get in Touch to get the complete white paper
This white paper is based on our State of Art (SOA) Analysis of “Solar Paint Technology: Innovations, Challenges, and Market Potential”.
Note: The SOA has been put through Notebook LLM to draft the report in White Paper Format. We have removed some of the links from references so as to not get penalized by google webmaster. To read the research papers with no links, copy the whole reference and put it in the google search.